Effect of pharmacogenetic variations on praziquantel plasma ... - Nature.com
Abstract
School-based mass drug administration (MDA) of Praziquantel (PZQ) is the global intervention strategy for elimination of schistosomiasis. Genetic variations in drug metabolizing enzymes and transporter proteins influences drug exposure and treatment outcomes, but data on PZQ pharmacokinetics and safety outcomes are scarce. We investigated the effect of pharmacogenetics variations on PZQ plasma concentrations and safety outcomes among 462 Rwandan schoolchildren who received single dose PZQ and albendazole in MDA. Genotyping for common functional variant alleles CYP3A4*1B, CYP3A5 (*3, *6, *7), CYP2C19 (*2, *3, *17), CYP2C9 (*2, *3) and CYP2J2*7 were done. Plasma concentration of PZQ, cis-4-OH-PZQ and trans-4-OH-PZQ were measured using LC/MS/MS. Active safety monitoring was done on days 1, 2, and 7 post-MDA. CYP2C9 and CYP2C19 genotypes were significantly associated with PZQ plasma concentrations and its cis- and trans-4-OH-PZQ/PZQ metabolic ratios (MR). CYP2C9*2 and CYP2C9*3 carriers had significantly higher PZQ concentration (p = 0.02), lower trans-4-OH-PZQ/PZQ (p < 0.001), and cis-4-OH-PZQ/PZQ (p = 0.02) MR. CYP2C19 (*2, *3) carriers had significantly higher plasma PZQ concentration than CYP2C19 *1/*1 and CYP2C19 *17 carriers (*1/*17 or *17/*17) (p < 0.001). CYP3A4 was significantly associated with cis-4-OH-PZQ MR (p = 0.04). Lower cis-4-OH-PZQ/PZQ MR (p < 0.0001) was a predictor of MDA-associated adverse events, but no significant association with genotypes were found. In conclusion, CYP2C9 and CYP2C19 genotypes significantly influence the plasma PZQ concentration and its MR. Lower cis-4-OH-PZQ/PZQ MR is significant predictor of adverse events following MDA.
Introduction
Praziquantel (PZQ) is the only World Health Organization (WHO) approved drug for the treatment and prevention of Schistosoma haematobium (urogenital schistosomiasis) and Schistosoma mansoni (intestinal schistosomiasis) currently1. Schistosomiasis is among the most prevalent neglected tropical diseases and public health problem in many parts of the world particularly in sub-Saharan Africa (SSA)2. More than 90% of all schistosomiasis cases are from SSA3. Approximately 20 million people suffer from schistosomiasis related complications and the infection causes up to 280,000 deaths annually4,5. The WHO recommends preventive chemotherapy of PZQ as a global intervention strategy to reduce transmission, control and eliminate schistosomiasis as a public health problem in endemic countries5. According to the WHO, in 2019, it was estimated that 236.6 million people required preventive treatment for schistosomiasis and more than 105.4 million people were reportedly treated2.
In settings where both schistosomiasis and soil transmitted helminths are endemic, single dose 40 mg/kg body weight of PZQ is co-administered with albendazole during mass drug administration (MDA)6. School children are higher risk of schistosomiasis and STH infections, hence the target population for preventive chemotherapy7,8. Although, mass PZQ administration played a significant role in reducing disease-associated morbidity and mortality, the disease remains endemic in many countries in SSA including Rwanda9,10,11. In Rwanda, transmission of intestinal schistosomiasis is prevalent, and children aged 5–10 years are the most affected8,12. Although mass PZQ and albendazole co-administration is generally safe, approximately 20% of children in Rwanda experienced mild to severe adverse events post MDA13.
Studies on other infectious diseases including malaria, HIV and Tuberculosis found that pharmacogenetic variation influences plasma drug exposure and treatment outcomes including safety14,15,16,17,18. Pharmacogenetic variations in drug metabolizing enzymes and transporter proteins relevant for PZQ disposition may have an impact on plasma concentration and treatment outcomes such as safety. PZQ is primarily metabolized by CYP450 enzymes, including CYP3A4, CYP3A5, CYP2C19 and CYP2C919 to produce several metabolites, including 4-OH-PZQ (trans- and cis-), a major metabolite of PZQ in human20. Most of these enzymes relevant for PZQ disposition are genetically polymorphic, displaying interindividual variability in enzyme activity21. The change in enzyme activity due to pharmacogenetic variations may cause inter-individual variability in plasma drug exposure and safety outcome (treatment-associated adverse events)21. Previous pharmacokinetics and pharmacodynamic studies have indicated that high plasma drug exposure may increase the risk of adverse events15,17,22. Therefore, genetic variations in CYP enzymes relevant for PZQ biotransformation may affect both PZQ plasma exposure and safety20. To date, few studies have investigated the role of pharmacogenetics on the pharmacokinetics of PZQ. Studies investigating the influence of genetic variations on PZQ plasma concentration are lacking23. To the best of our knowledge, only two studies investigated the pharmacogenetics variations of PZQ and its relevance on plasma concentration and schistosomiasis treatment outcomes among Schistosomiasis infected children in Tanzania and Zimbabwe20,24. The authors reported relevance of CYP2C19 genotype for PZQ concentration and trans-4-OH-PZQ/PZQ ratio and a borderline association between CYP3A5 genotype and treatment-associated adverse events20. More studies are needed to characterize the importance of pharmacogenetic variations for PZQ plasma exposure and treatment outcome since the populations differ partly due to wide genetic diversity among black African populations17,25. Our study is the first to investigate the effect of pharmacogenetics on PZQ concentration and safety outcome among children who received PZQ as part of MDA. Additionally, the effect of CYP2J2 genotype on PZQ concentration and the effect of pharmacogenetic variation on cis-4-OH-PZQ concentration and its metabolic ratio (MR), cis-4-OH-PZQ/PZQ have not been investigated.
The utility of pharmacogenetic data to improve treatment outcomes has recently been intensified in Africa26. However, the influence of pharmacogenetic variations on the pharmacokinetics and safety outcome of drugs used in MDA campaigns remains to be investigated. Even though it is a challenge to implement individualized treatment during MDA, understanding how genetic variations impact the pharmacokinetics and treatment associated adverse events of drugs used in MDA is essential for improving treatment outcomes in the future14,20,27. Therefore, the objective of this study was to investigate the effect of pharmacogenetics variations on PZQ plasma concentrations and safety outcomes among school children who received PZQ co-administered with albendazole in Rwanda.
Materials and methods
Study design, setting and population
This pharmacogenetics, pharmacokinetics, and pharmacodynamics (safety) prospective cohort study included 462 school children aged 5–15 years attending six schools in three districts located around the belt of Lake Kivu, namely Rubavu, Nyamasheke, and Rusizi in the Western Province of Rwanda. The three districts are endemic for intestinal schistosomiasis and soil transmitted helminths. This study received ethical approval from Rwandan National Ethics Committee and Karolinska Institutet, and was conducted in accordance with the principles outlined in the Declaration of Helsinki.
Data including sociodemographic, anthropometrics (height, weight) and pre-MDA symptoms for safety assessment were collected using a case record form administration through interviews. Anthropometric measurements, children's body weight was measured in kilograms (kg) and height was measured in centimeters (cm), and then converted to body mass index (BMI)-for-age Z score (BAZ) and height-for-age Z score (HAZ), respectively, using WHO Anthro-Plus software for school-age children28. Children who had HAZ and BAZ scores < 2 standard deviations (SD) were considered stunted and wasted/thin, respectively.
Preventive chemotherapy and adverse event monitoring
All participants were interviewed for any pre-existing clinical symptoms (pre-MDA event), such as fever, loss of appetite, dizziness or fainting, confusion, drowsiness, headache, cough, difficulty in breathing, nausea, vomiting, diarrhea, stomach pain, itching, rash, and any other symptoms. Study participants received single dose albendazole and PZQ in MDA campaigns as preventive chemotherapy provided through the Rwandan national NTD program. All children were informed to eat food before coming to school to participate in the MDA campaign. Number of PZQ tablets was based on height of children (≥ 94 cm dose pole, designed to deliver a dose of at least 40 mg/kg) and albendazole 400 mg following the national and WHO MDA guidelines6,29. The Rwanda NTD public health program of the Ministry of Health provided and co-administered PZQ and albendazole as preventive chemotherapy to prevent transmission and control of schistosomiasis and STH.
Following MDA, study participants were actively monitored to record MDA-related adverse events (AEs) on days 1, 2, and 7 post MDA13. Collected data were entered into an electronic database using tablets and cleaned before statistical analysis. To distinguish between pre-existing clinical symptoms and treatment-related adverse events (AEs) following PZQ and albendazole MDA, events reported by each study participant before and after MDA were cross-checked and verified13. Treatment-associated adverse events (AEs) were defined as any medical event (sign or symptom) that occurred after drug intake (post-MDA) and the same type of events were not reported prior to the administration of the drug (pre-MDA)13,30,31.
Quantification of praziquantel, cis-4-hydroxy-praziquantel and trans-4-hydroxy-praziquantel plasma concentrations
Reference standards such as racemic PZQ, trans-4-OH-PZQ, and cis-4-OH-PZQ and their respective internal standards rac-PZQ-d11, trans-4-OH-PZQ-d5, and cis-4-OH-PZQ-d5 were purchased from Toronto Research Chemicals (Toronto, Ontario, Canada). Acetonitrile, methanol, and formic acid of mass spectrometry (MS) grade were purchased from Merck (Darmstadt, Germany). Ultra-pure MilliQ water was prepared using a Milli-Q water purification system (Merck Millipore, Massachusetts, USA). Blank plasma was supplied by the blood bank of the Karolinska University Hospital Huddinge (Stockholm, Sweden).
Whole blood sample was collected 2 h post MDA from all 462 study participants in heparinized tubes and immediately centrifuged at 1000 rpm for 10 min and plasma aliquot was kept in − 80 °C freezer until analysis. The plasma concertation of PZQ, trans-4-OH-PZQ and cis-4-OH-PZQ using Ultra high Performance Liquid Chromatography tandem–Mass Spectrometry (UPLC-MS/MS) as described previously20. In brief, 100 µL of plasma sample went through protein precipitation with 300 µL of internal standards solution containing 50 ng/mL each of rac-PZQ-d11, trans-4-OH-PZQ-d5, cis-4-OH-PZQ-d5 in a 50:50 mixture (v:v) of acetonitrile:methanol. The mixture was vortexed for 3 min and centrifuged for 20 min at 3220 g at 4 °C. Thereafter, 75 µL of the supernatant was diluted with 75 µL MilliQ water, and 5 µL of the mixture was injected into the UPLC-MS/MS system. Standards and quality control (QC) samples were prepared in the same manner by adding 10µL standard and QC 10× concentrated solutions to 90 µL blank plasma.
The calibration curves were constructed within the range of 2.4 to 2500 ng/mL for PZQ and cis-4-OH-PZQ. For trans-4-OH-PZQ, the range of the calibration curve was constructed within 24 to 25,000 ng/mL. About 7–9 calibration points were injected twice before the samples and once after. The QC samples were injected for every 20 samples. Quantification of the analytes was performed using the analyte to internal standard integrated peak area ratio with the MassLynx application manager TargetLynx (Waters). Trans-4-OH-PZQ d5 was used as internal standard also for cis-4-OH-PZQ since their retention times were similar. Quality control samples at 9.8, 78.1, and 1250 ng/mL were injected at regular intervals during each analysis.
Genotyping for CYP3A4, CYP3A5, CYP2C19, CYP2C9 and CYP2J2
Genomic DNA was extracted from whole blood using the QIAamp DNA Midi Kit (Qiagen GmbH, Germany) as per the manufacturer's instruction. Genotyping for CYP3A4*1B, CYP3A5*3, CYP3A5*6, CYP3A5*7, CYP2C19*2, CYP2C19*3, CYP2C19*17, CYP2C9*2, CYP2C9*3, and CYP2J2*7 was done as previously described14,20. Briefly, genotyping was performed using TaqMan® drug metabolism genotyping assay reagents for allelic discrimination (Applied Biosystems Genotyping Assays) with the following ID numbers for each SNP: C__11711730_20 for CYP3A4*1B (− 392A>G, rs2740574), C__26201809_30 for CYP3A5*3 (c.6986A4G, rs776746), C__30203950_10 for CYP3A5*6 (g.14690G4A, rs10264272), C__32287188_10 for CYP3A5*7 (g.27131_27132insT rs41303343), C__25986767_70 for CYP2C19*2 (rs4244285), C__2,7861809_10 for CYP2C19*3 (rs4986893), C__469857_10 for CYP2C19*17 (rs12248560), C__25625805_10 for CYP2C9*2 (rs1799853), C__27104892_10 for CYP2C9*3 (rs1057910) and C__9581699_80 for CYP2J2*7 (rs890293). Genotyping was done using 7500 Fast Real-Time PCR (Applied Biosystems, United States). The final volume for each reaction was 10 μL, consisting of 1 μL genomic DNA and 9 μL TaqMan fast advanced master mix (Applied Biosystems, Waltham, MA, United States), DNA/RNA free water and TaqMan drug metabolism genotyping assays mix (Applied Biosystems). The PCR consisted of an initial step at 60 °C for 30 s, hold stage at 95 °C for 10 min and PCR stage for 40 cycles step 1 with 95 °C for 15 and step 2 with 60 °C for 1 min and after read stage with 60 °C for 30 s.
Statistical analysis
Socio-demographic characteristics were summarized as proportions for categorical data and median (range or Interquartile range- IQR). The CYP3A4 (*1/*1 or *1B carrier), CYP3A5 (*1/*1 or *3, *6 *7 carriers), CYP2C9 (*1/*1 or *2, *3 carriers) and CYP2J2 (*1/*1 or *7 carriers) genotypes were dichotomized as variant allele carriers and non-carriers (wild type). CYP2C19 genotype was categorized as Ultrarapid Metabolizer (*1/*17 and *17/*17), Extensive Metabolizers (*1/*1), and intermediate or poor metabolizers (*2, *3 carriers). Chi-square test was used to compare the genotype and allele frequencies between the observed and expected according to the Hardy–Weinberg equilibrium.
Plasma concentration of PZQ and its MRs were transformed to log10 values before statistical analysis. Independent t-test or one-way ANOVA was used to compare the geometric means of PZQ, cis-4-OH-PZQ/PZQ and trans-4-OH-PZQ/PZQ MR concentrations between genotypes. A univariate followed by multivariate linear regression analysis were used to identify the predictors of PZQ plasma concentrations. Independent samples t-test was used to compare the geometric means of PZQ and its trans and cis-4-OH MRs among those who experienced any adverse event and those who did not experience adverse events. Chi-square or fisher's exact tests was used to test for associations between adverse events following MDA and genotypes. Univariate and multivariate log binomial regression was used to quantify the effect of association between the different genotypes and adverse event.
Ethical approval
This study received ethical approval from the Rwandan National Ethics Committee (Review Approval Notice No. 0064/RNEC/2019) and Stockholm Ethics Committee, Sweden (Ref.No. 2020-00845). Prior to enrolment, participants and their parents or legal guardians received information about the study. For participants ≤ 12 years of age, verbal and written informed consent was obtained from their parent or guardian, and for participants > 12 years of age, verbal and written informed consent was obtained from the parent or guardian and assent was obtained from the study participant.
Results
Sociodemographic and nutritional status of study participants
A total of 462 school children who received PZQ and albendazole MDA were enrolled in this study, of whom 50.4% were females. The median age (interquartile) of the study cohort was 12 (10–13) years. The median age (interquartile) of 37. The median weight and height were 32 kg (IQR = 27–39) and 137 cm (IQR = 129–146). The proportion of children who were stunted and wasted were 4% and 3.0% respectively.
Genotype and allele frequencies
The genotype and allele frequencies for CYP3A4*1B, CYP3A5 (*3, *6, *7), CYP2C19 (*2, *3, *17), CYP2C9 (*2, *3) and CYP2J2 (*7) among Rwandan children is presented in Table 1. There were no significant differences between the observed and expected genotypes frequencies according to the Hardy Weinberg Equilibrium. The most frequent variant was CYP3A4*1B (72.0%), followed by CYP3A5*6 (20.0%) and CYP3A5*3 (18.2%). CYP2C9*2 and CYP2C9*3 alleles occurred at the lowest frequency (0.2%).
Effect of genotypes on plasma praziquantel concentrations
The overall geometric means ± SD concentrations of PZQ, trans-4-OH-PZQ and cis-4-OH-PZQ in the study population were 318.4 ± 4.1, 8770.0 ± 2.2 and 571.5 ± 2.8 ng/mL, respectively. The overall geometric means ± SD concentrations of the PZQ MRs namely trans-4-OH-PZQ/PZQ and cis-4-OH-PZQ/PZQ were 27.5 ± 2.7 and 1.8 ± 2.9 ng/mL, respectively.
Comparison of PZQ, trans-4-OH-PZQ/PZQ and cis-4-OH-PZQ/PZQ concentration between the different genotypes are presented in Table 2. CYP2C9 *2, *3 carriers had significantly higher PZQ concentration and lower trans-4-OH-PZQ and cis-4-OH-PZQ MRs compared to children with CYP2C9*1/*1 genotype. CYP2C19 genotype was significantly associated with PZQ and cis-4-OH-PZQ/PZQ concentrations. Children with CYP2C19 *1/*17 or *17/*17 (ultra-rapid metabolizers) had the lowest PZQ concentration and children with *2, *3 carriers (intermediate and poor metabolizers) had the lowest PZQ concentration. Conversely the trans-4-OH-PZQ and cis-4-OH-PZQ MRs where highest among CYP2C19 *1/*17 or *17/*17, and those who were *2, *3 carriers had the lowest MRs. CYP3A4 was also associated with cis MR, CYP3A4*1B carriers had a higher cis-4-OH-PZQ/PZQ MR compared with those who carried than CYP3A4*1/*1 genotypes. No significant effect of CYP3A5 and CYP2J2*7 genotypes on PZQ and its MRs was observed.
Predictors of praziquantel plasma concentrations
Factors associated with plasma PZQ concentration is shown in Table 3. In the univariate linear regression analysis, age, weight, height, and genotypes CYP2C9 and CYP2C19 were significant predictors of PZQ concentration. In the multivariate model, after adjusting for age, height, weight and CYP2C9, CYP2C19 genotypes remained significantly associated with plasma PZQ concentration. CYP2C19*2 and CYP2C19*3 carriers (intermediate and poor metabolizers) had a mean increase concentration of 0.185 ng/mL (95% CI 0.045–0.326) compared to those with CYP2C19*1/*1 (extensive metabolizers) (p value = 0.01). Ultrarapid metabolizers (CYP2C19 *1/*17 or *17/*17) had a lower mean PZQ concentration of 0.08 ng/mL (95% CI − 0.208 to 0.048) compared to extensive metabolizers but this was not statistically significant (p = 0.22). After adjusting for CYP2C19, age, height and weight, carriers of CYP2C9*2 or CYP2C9*3 had an increased mean PZQ concentration of 0.54 ng/mL (95% CI − 0.056 to 1.134, p = 0.08) compared to CYP2C9*1/*1 genotypes.
Predictors of praziquantel metabolic ratios
Predictors for the PZQ MR concentrations namely trans- and cis-4-OH-PZQ/PZQ are presented in Table 4. Age, height, weight, CYP2C9 and CYP2C19 were crude significant predictors of both trans and cis-4-OH-PZQ/PZQ MR. CYP3A4 was also a crude predictor for cis-4-OH-PZQ/PZQ. In the adjusted model, statistically significant predictors for trans-4-OH-PZQ/PZQ MR included age, CYP2C9 and CYP2C19. For every one-year increase in age, the mean concentration of trans-4-OH-PZQ/PZQ MR decrease by 0.034 (p value 0.01; 95% CI − 0.061 to − 0.007). CYP2C9 *2 and *3 carriers had significantly lower trans-4-OH-PZQ/PZQ mean MR compared to those with CYP2C9 *1/*1 genotypes. The mean trans MR was lower among CYP2C19 *2 and *3 carriers (p < 0.001) compared to non-carriers of the variant alleles. Children who carried CYP2C19 *1/*17 or *17/*17 had a significantly higher mean trans-4-OH-PZQ/PZQ MR of 0.136 (p-value < 0.001; 95% CI 0.049–0.223) compared to children who were *1/*1. Cis-4-OH-PZQ/PZQ concentration was significantly associated with CYP3A4 and CYP2C19 genotypes after adjusting for selected variables. CYP3A4*1B carriers had a mean increased mean cis MR of 0.165 (p value 0.04; 95% CI 0.010–0.320) compared to with CYP3A4 *1/*1. CYP2C19 *2, *3 carriers had a lower mean cis-4-OH-PZQ/PZQ MR compared to CYP2C19 *1/*1 (p value < 0.01). There was a borderline association between CYP2C9 genotype and cis-4-OH-PZQ/PZQ MR, in comparison with children who were CYP2C9 *1/*1, the mean MR was lower by 0.425 (p value = 0.06; 95% CI − 0.875 to 0.025) among children who carried CYP2C9 *2, *3 alleles.
Association of Praziquantel concentrations and genotype with MDA-associated adverse events
A total of 436 children completed the safety 7-day post-MDA follow-up, of whom 36.0% (31.5–40.7%) reported at least one type of MDA-associated AE. The total number of AEs reported was 348, of which most occurred on the first day of MDA (83.0%) followed by day 2 (13.5%), and during days 3–7 of post MDA (3.5%). The severity of reported AEs was graded as mild, moderate, or severe, following the Common Terminology Criteria for Adverse Events (CTCAE) version 5.032. The proportion of mild and moderate AEs were 92.2% (number of AEs = 321) and 7.5% (number of AEs = 26), respectively. Only one AE (0.3%) was severe. No potentially life-threatening or disabling AEs or death was observed. The cumulative incidences of specific type of adverse events are presented in Fig. 1. The most common AEs were a headache, dizziness, nausea, and abdominal pain.
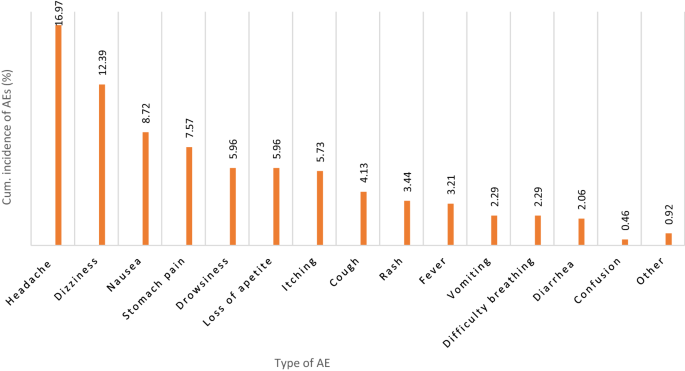
Type and cumulative incidence of MDA associated adverse events (AEs) during the 7-day follow-up.
Children who experienced MDA-associated adverse had higher geometric mean PZQ plasma concentration compared to children who did not, but the difference was not statistically significant (p = 0.15) as shown in Table 5. The geometric mean cis-4-OH-PZQ/PZQ MR was significantly lower among those who experienced adverse events compared to those who did not (p < 0.0001). There was also difference in crude and adjusted mean cis metabolic ratios among those children who experience adverse events compared to those who did not. After adjusting for age, weight and height, children who experienced adverse events had a mean decrease cis-4-OH-PZQ/PZQ concentration of 0.131 (p < 0.01; 95% CI − 0.223 to − 0.039) compared to children who did not experience adverse events. There was no statistically significant difference in trans-4-OH-PZQ/PZQ MR concentration between children who experienced adverse events compared to those who did not. Univariate followed by multivariate logistic regression analysis indicated no association between CYP3A4, CYP3A5, CYP2C9, CYP2C19 and CYP2J2 genotypes and experiencing MDA-associated adverse events.
Discussion
PZQ is metabolized by polymorphic enzymes and its plasma exposure and treatment outcome display wide inter-individual variability. Recent studies highlighted the need for more comprehensive studies of the PZQ metabolic pathway and PZQ pharmacogenetic studies in humans20,23. In this study, we investigated the effect of pharmacogenetic variations on PZQ pharmacokinetics and MDA-associated adverse events as well as the effect of pharmacokinetics on safety outcomes among school children who received praziquantel and albendazole preventive chemotherapy in Rwanda. Our major finding indicates that CYP2C19 and CYP2C9 genotypes as significant predictors of plasma PZQ concentration and its MRs (trans- and -cis-4-OH-PZQ/PZQ MR). Furthermore cis-4-OH-PZQ/PZQ MR concentration was significantly associated with CYP3A4*1B genotype. Another key finding was that cis-4-OH-PZQ/PZQ concentration was significantly associated with safety outcome (MDA-associated adverse event). There was no association between genotypes and adverse event following MDA.
PZQ is a racemic mixture R- and S-PZQ and is metabolized mainly by cytochrome P450 enzymes in the liver, specifically CYP2C19, CYP2C9, CYP3A4, CYP1A2, and CYP2D633,34. We found significant associations between CYP2C19 and CYP2C9 genotypes with PZQ concentration and trans and cis-4-OH-PZQ MRs. Higher PZQ plasma concentration was observed in children with CYP2C19 defective variant alleles compared to children carrying CYP2C19 *1/*1 and CYP2C19 *1/*17 or *17/*17 (ultra-rapid metabolizers). The mean of trans and cis-4-OH-PZQ/PZQ MRs were highest among ultra-rapid metabolizers (CYP2C19 *1/*17 or *17/*17) compared to those with carriers of CYP2C19 defective variant alleles indicating that ultra-rapid metabolizers produce more metabolites compared to the other phenotypes. Although our study population received PZQ and albendazole as preventive chemotherapy without prior screening for the diseases, our finding is in line with a recent study among Schistosoma mansoni infected Tanzanian children who received PZQ therapy20. Our finding may indicate that CYP2C19 is a major metabolic pathway for the formation of trans- and -cis-4-OH-PZQ metabolites. Li et. al., found that CYP1A2 and CYP2C19 are the main enzymes responsible for metabolizing PZQ to its major metabolite, 4-OH-PZQ35. R-PZQ reportedly produces higher concentrations of cis- and trans-4-OH-PZQ than its racemate, S-PZQ36,37. A recent study using recombinant and human liver microsomes reported that metabolism of R-PZQ was mainly catalyzed by CYP1A2 and CYP2C19, whereas metabolism of S-PZQ was mainly by CYP2C19 and CYP3A437. Though polymorphic, functional defective variant alleles of CYP1A2 are very rare in humans38,39. Hence the effect of CYP1A2 genetic variations in determining variability in plasma PZQ exposure could be minor.
Furthermore, we found significantly higher concentration of PZQ among children CYP2C9*2 or*3 carriers compared to those with CYP2C9 *1/*1. In the multivariable linear regression, after adjusting for potential confounders, the association between CYP2C9 genotype was only significantly associated with trans-4-OH-PZQ/PZQ and cis-4-OH-PZQ/PZQ MRs and borderline associated with PZQ concentration. Our finding further supports that PZQ is mainly metabolized by CYP2C19 and to a lesser extent by CYP2C935,37. Furthermore, a significant association between CYP3A4 genotype and cis-4-OH-PZQ/PZQ but not PZQ concentration or trans MR was found. CYP3A4*1B carriers had significantly higher cis-4-OH-PZQ/PZQ MR compared to those with CYP3A4*1/*1. The effect of CYP3A4*1B on lumefantrine plasma exposure is reported previously14. Our finding indicates that compared to CYP2C19 and CYP2C9, the impact CYP3A4 and 3A5 genotype appears to be minor in determining variability in plasma PZQ exposure. The 4-hydroxylation of PZQ is reported to be the major metabolic pathway of PZQ, as evidenced by larger quantities of 4-OH-PZQ produced40.
In this study, we did not investigate the effect of CYP1A1/2 and CYP2D6 genotypes even though few studies reported their involvement in the metabolism of PZQ33,34,37. This can be considered as our study limitation, although functional CYP1A2 defective variant alleles are rare38. More studies are needed to investigate the influence of those genotypes on PZQ concentration especially when albendazole and PZQ are co-administered, it was reported that albendazole moderately inhibits the CYP1A2 enzyme41. Furthermore, coadministration of PZQ and albendazole showed that the exposure of R-PZQ increased by 64.77% (AUC increased from 0.52 to 0.86 μg/mL h) but there was no significant difference in the AUC of S-PZQ42, this can because one or more enzymes are involved in PZQ and albendazole metabolism. This may improve the therapeutic outcome because of higher concentration of R-PZQ, the pharmacologically active enantiomer. On the other hand, this increase in R-PZQ concentration may affect safety even though, S-PZQ is the main contributor of PZQ associated side effects. Therefore, further studies are needed to evaluate treatment outcomes of the combination of PZQ and albendazole.
Non-genetic factors that influenced PZQ exposure in our study was age. We found age as a predictor of trans-4-OH MR, the main metabolite of PZQ in humans. This could be because of the rapid first pass effect (metabolism) of R-PZQ. Differences in the first pass effect based on age has been previously reported43,44.
Our finding indicates the relevance of plasma PZQ exposure and its MRs for MDA-associated adverse events. Though not significant, children who experienced adverse events had a higher mean plasma PZQ compared to those who did not. Interestingly there was a significant difference in mean cis MR among those the experienced adverse events compared to those who did not, this may indicate that those who experienced adverse events had a higher concentration of PZQ. Previous studies reported S-PZQ, the non-therapeutically active enantiomer, as the main contributor of the bitter taste of the drug and side effects such as nausea and vomiting45,46,47.
We found no significant association between the CYP3A4, CYP3A5, CYP2C9, CYP2C19 and CYP2J2 and MDA-associated adverse events safety treatment outcome. However, a previous study reported significantly higher incidence of adverse events among carriers of CYP3A5 defective variant allele (67.0%) compared to those with CYP3A5 *1/*1 (33.0%)20. Any association of CYP3A genotype with adverse events could explained due to its relevance for S-PZQ metabolic pathway. CYP3A is the main enzyme that metabolizes S-PZQ19,37, which is responsible for the unpleasant taste of PZQ causing nausea and vomiting. As we quantified the racemate PZQ and not individual enantiomers, any association of genotype with enantiomers-specific adverse events could not be explored in our study.
Therefore, we recommend more studies investigating the association of CYP3A genotypes with S-PZQ plasma exposure and safety outcomes post PZQ exposure.
The study limitations include the following: We investigated the effects of CYP genotype on plasma concentration of praziquantel and metabolic ratio of the main metabolites 4-OH-PZQ, specifically trans-4-OH-PZQ. Since PZQ is administered as a racemic mixture of the two enantiomers, we measured total plasma PZQ concentration, but not R-PZQ and S-PZQ separately. Hence the effect of genotype on the enantiomer specific metabolism of PZQ were not assessed. Furthermore, we quantified the plasma concentrations of trans and cis-4-OH-PZQ, the main metabolites of R-PZQ, which is the therapeutically active form of PZQ33,34. Recent studies reported the importance of CYP3A for the metabolism of S‐PZQ to X‐OH‐PZQ40,48. As we did not measure X-OH-PZQ, the role of genotype on the metabolism of S-PZQ and its metabolic ratio were not explored.
Conclusion
Our study highlights the importance of pharmacogenetic variation for PZQ pharmacokinetics and association of pharmacokinetics with safety outcomes. CYP2C19 and CYP2C9 were associated with plasma PZQ concentrations and cis- and trans-4-OH-PZQ MRs in Rwandan children. CYP3A4 was only associated with the cis-4-OH-PZQ MR. Those who experienced adverse events had a significantly lower mean cis-4-OH-PZQ MR compared to those who did not. There was no association between CYP3A4, CYP3A5, CYP2C9, CYP2C19 and CYP2J2 genotypes and safety outcome. More studies are needed to evaluate the effect of pharmacogenetics on pharmacokinetics and pharmacodynamic especially among those who carry defective variant alleles of CYP2C9 and CYP2C19.
Data availability
All data generated or analysed in this study are included in this published article, and the datasets are available from the corresponding author upon reasonable request owing to privacy and ethical restrictions from the authors.
References
World Health Organization. Investing to Overcome the Global Impact of Neglected Tropical Diseases, Third WHO Report on Neglected Tropical Diseases (WHO, 2015).
World Health Organization. Schistosomiasis. https://www.who.int/en/news-room/fact-sheets/detail/schistosomiasis (Accessed 5 August 2022).
Mazigo, H. D. Participatory integrated control strategies and elimination of schistosomiasis in sub-Saharan Africa. Lancet Glob. Health 7, e998–e999. https://doi.org/10.1016/s2214-109x(19)30271-2 (2019).
Hotez, P. J. et al. The global burden of disease study 2010: Interpretation and implications for the neglected tropical diseases. PLoS Negl. Trop. Dis. 8, e2865. https://doi.org/10.1371/journal.pntd.0002865 (2014).
Fenwick, A. et al. The Schistosomiasis Control Initiative (SCI): Rationale, development and implementation from 2002–2008. Parasitology 136, 1719–1730. https://doi.org/10.1017/s0031182009990400 (2009).
World Health Organization. Preventive Chemotherapy in Human Helminthiasis: Coordinated Use of Anthelminthic Drugs in Control Interventions: A Manual for Health Professionals and Programme Managers (WHO, 2006).
Adenowo, A. F., Oyinloye, B. E., Ogunyinka, B. I. & Kappo, A. P. Impact of human schistosomiasis in sub-Saharan Africa. Braz. J. Infect. Dis. 19, 196–205. https://doi.org/10.1016/j.bjid.2014.11.004 (2015).
Rujeni, N., Morona, D., Ruberanziza, E. & Mazigo, H. D. Schistosomiasis and soil-transmitted helminthiasis in Rwanda: An update on their epidemiology and control. Infect. Dis. Poverty 6, 8. https://doi.org/10.1186/s40249-016-0212-z (2017).
Gebreyesus, T. D. et al. Prevalence, intensity, and correlates of schistosomiasis and soil-transmitted helminth infections after five rounds of preventive chemotherapy among school children in southern Ethiopia. Pathogens 9, 920 (2020).
Mnkugwe, R. H., Minzi, O. S., Kinung'hi, S. M., Kamuhabwa, A. A. & Aklillu, E. Prevalence and correlates of intestinal schistosomiasis infection among school-aged children in North-Western Tanzania. PLoS ONE 15, e0228770. https://doi.org/10.1371/journal.pone.0228770 (2020).
Nyandwi, E., Veldkamp, A., Amer, S., Karema, C. & Umulisa, I. Schistosomiasis mansoni incidence data in Rwanda can improve prevalence assessments, by providing high-resolution hotspot and risk factors identification. BMC Public Health 17, 845. https://doi.org/10.1186/s12889-017-4816-4 (2017).
Hanotier, J. & Gigase, P. L. Note on a new focus of schistosomiasis (S. mansoni) in Rwanda. Ann. de la Soc. belge de Med. Trop. 61, 93–98 (1981).
Kabatende, J. et al. Safety of praziquantel and albendazole coadministration for the control and elimination of schistosomiasis and soil-transmitted helminths among children in Rwanda: An active surveillance study. Drug Saf. https://doi.org/10.1007/s40264-022-01201-3 (2022).
Mutagonda, R. F. et al. Effect of pharmacogenetics on plasma lumefantrine pharmacokinetics and malaria treatment outcome in pregnant women. Malar. J. 16, 267. https://doi.org/10.1186/s12936-017-1914-9 (2017).
Comments
Post a Comment